Top climate change papers on tropical forests
Our AI creates an annotated bibliography from papers with the most supporting citations from scite.ai
Scite.ai is a fantastic discovery and literature validation service. Here, we take a feed of climate change papers available via the Scite dashboard, and our machine-reading engine then summarises the papers on tropical forests that have the most supporting citations.
Photo by Vladimir Soares on Unsplash
The amount of water available to sustain evergreen vegetation during the dry season in tropical rainforests is determined by the level of annual rainfall, an analysis of satellite data suggests.
In ‘Photosynthetic seasonality of global tropical forests constrained by hydroclimate’, Kaiyu Guan et al. (2015) reported that the response of tropical forests to droughts is highly uncertain. They find that above an annual rainfall threshold of approximately 2,000 mm yr−1, the evergreen state is sustained during the dry season in tropical rainforests worldwide. They conclude that water availability exerts a first-order control on vegetation seasonality in tropical forests globally. Canopy photosynthesis of some tropical forests can decline, whereas in others it can be maintained at the same or a higher level than during the wet season.
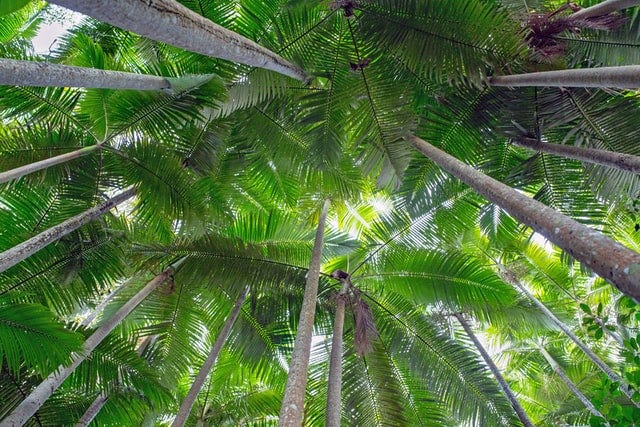
Trees in tropical forests are more vulnerable to drought than trees in other parts of the world, according to an analysis of data from 119 monitoring plots in 10 countries.
A research group from the Ecology and Global Change led by Oliver Phillips (2010) report that terrestrial life thrives where both warmth and water supply are greatest. In the wet lowland tropics, biodiversity, productivity and carbon stocks all tend to reach their greatest values. Phillips and colleagues assembled available data on tropical forest tree stem mortality before, during, and after recent drought events. The stem mortality of tropical trees increased with the intensity of dry season moisture stress. There is some evidence for lagged impacts of drought, with mortality rates remaining elevated 2 yr after the meteorological event is over. These findings indicate that repeated droughts would shift the functional composition of tropical forests toward smaller, denser-wooded trees.
An additional 16 models were considered for Amazonia – excluding the experimental droughts – and a further 48 for the whole tropics, Amazonia, and Borneo. The group then selected the best models for each of the data set on the basis of adjusted R2 and information criterion statistics. Some of the conclusions appear to offer an alternative view to prior work in this area: “In such events, drought adaptations would carry a severe selective penalty in reduced competitive vigour. But the converse expectation is also plausible – an excess moisture deficit should have the greatest impacts in normally drier forests. Here there is a greater risk of crossing a biome threshold to savanna climate,” Phillips said. They suggest that they included literature that published mortality rates for the same permanent plots through pre-drought and drought intervals. They included additional studies from Borneo where extreme droughts affected the forest in 1982–1983 and 1997–1998. Forests growing in the most extreme soil conditions – white sands or frequently water-logged soils – were excluded.
A review of the evidence for the existence of Seasonally Dry Tropical Forests (SDTFs) in lowland cis‐Andean eastern‐central South America.
Werneck and colleagues (2010) studied revisiting the historical distribution of Seasonally Dry Tropical Forests. The LGM climate was probably too dry and cold to support large tracts of SDTF. Expansions ofSDTFs are proposed to have occupied the southern portion of Caatinga nucleus more recently during the early–middle Holocene transition. The authors discuss the possibility that SDTFs may experience future expansion under changing climate scenarios. They first developed georeferenced maps depicting the current distributional extent of SDTFs under two distinct definitions. The team generated occurrence datasets which were used with current and past bioclimatic variables to predict SDTF occurrence. They obtained historical stability maps by overlapping the presence/absence projections of each of three climatic scenarios.
The role of the emerged continental shelf on the Atlantic Forest biodiversity hotspot of eastern South America during glacial periods has been ignored in the literature.
In ‘Neotropical forest expansion during the last glacial period challenges refuge hypothesis’, Yuri Leite et al. (2016) reported that to test putative scenarios of population diversification, they implemented coalescent simulations in the Bayesian Serial SimCoal (BayeSSC) (60, 61), and they evaluated the fit of the empirical genetic data to distinct historical models. They evaluated the fit of the empirical genetic data to distinct historical models. Bottlenecks and expansions were first simulated for each period separately. Ne was estimated from theta, according to the following formula. They simulated a scenario of a single panmictic population without any demographic event throughout time, which was compared with three population subdivision models.
Five species of small mammals, three didelphid marsupials and two cricetid sigmodontine rodents, were included in this study. These small mammals are all forest specialist species, for which ecological data indicate a clear preference for forests.
Humid tropical forests are one of the most important sources of carbon in the Earth's atmosphere.
A research group led by Nicholas Bouskill of the Ecology Department (2012) studied how pre-exposure to drought increases the resistance of tropical forest soil bacterial communities to extended drought. Humid tropical forests are globally important carbon reservoirs, storing B25% of terrestrial organic C. Does exposure to prior stress confer increased resistance to subsequent perturbation? Here they reduced the quantity of precipitation throughfall to tropical forest soils in the Luquillo Mountains, Puerto Rico. Microbial communities within humid following year, the shelters were tropical rainforest soil may be more likely to adapt replaced over these original treatment plots.
Data and code are available from: http://www.nature.com/ismej.
Planting trees with more than one species increases the amount of carbon stored in the soil.
Yuanyuan Huang et al. (2018) used the additive partitioning method of Loreau & Hector to decompose net biodiversity effects (NEs) of productivity measures into complementarity (CEs) and selection effects (SEs). The team assessed the effects of trait and phylogenetic distances on different components of diversity effects of two-species mixtures with linear mixed-effects models. They report how stand-level productivity in the BEF-China experiment 3–7 years after planting was related to species richness. The group found significantly positive effects of the logarithm of tree species richness on both stand volume and annual stand volume increment of trees (F1, 89 = 5.26, P =.26).
The group manipulated tree species richness by planting more than 150,000 trees in plots with 1 to 16 species. Simulating multiple extinction scenarios, they found that richness strongly increased stand-level productivity. The researchers have provided data and code at: https://doi.pangaea.de/xxxxxxxxxxxxxx.
Carbon loss from deforestation in the Amazon region could account for up to 40% of greenhouse gas emissions in the region.
A research group at the Centro de Energia Nuclear na Agricultura; Universidade de São Paulo; Av. Centenário 303 led by Erika Berenguer (2014) reported on a large-scale field assessment of carbon stocks in human-modified tropical forests. Anthropogenic forest degradation is the reduction in the overall capacity of a forest to supply goods and services. The researchers present the largest field study to date detailing the effects of anthropogenic forest disturbance on the aboveground, dead wood, litter, and soil carbon pools. Trees between 2 and 10 cm DBH increased their average contribution to aboveground stocks by 119% and 38% in logged-and-burned forests, respectively. In tropical forests, the degree of degradation of carbon stocks depends on the type of disturbance, the intensity and frequency of disturbance events, and the time elapsed since their occurrence. The team show that forest disturbance, when resulting from a combination of both fire and logging, can severely alter forest structure.
Results were not sensitive to slope as the relationship between slope-corrected and uncorrected estimates of aboveground carbon yielded a R2 > 0.99. In total, they measured 70 293 stems of trees, palms, and lianas. The researchers’ findings potentially offer an alternative view to prior studies in this area: “Distance to edge acts as a proxy for a multitude of different effects that have negative impacts on plant biomass, such as increase in air temperature and wind disturbance. Given the ease of access, forests close to edges are more susceptible to relatively low-intensity and small-scale selective logging and understory fires,” Berenguer claimed.
Tropical forests are under increasing pressure from climate change.
Kenneth Feeley and colleagues (2011) report that tropical forests face many challenges, including natural climatic events, such as El Nino-associated droughts, and large-scale anthropogenic disturbances, including habitat loss and climate change. Feeley and colleagues investigated the nature and underlying causes of compositional change in a tropical forest using census data from the 50-ha Forest Dynamics Plot on Barro Colorado Island, Panama. Feeley and colleagues tested if species composition of the BCI tree community has shifted appreciably since the plot was first established. The mean rate of succession as measured by the change in the subplots’ average gap phase (GP) status through time did not differ significantly from zero.
A research team from the Department of Ecosystem and Conservation Sciences led by Alan Townsend (2011) reported in ‘Multi‐element regulation of the tropical forest carbon cycle’ that resolving the complex nature of tropical forest nutrient limitation – and incorporating such knowledge into predictive models – will be difficult. The authors do not even know which nutrient or nutrients limit NPP and C storage in most tropical forests. Techniques that can effectively integrate the extraordinary complexity of the tropical forest biome over large scales should greatly enhance the ability to monitor and predict the tropical C cycle. The understanding of how nutrients control the tropical carbon cycle remains far from complete. This knowledge gap arises from the marked complexity of the tropical forest biome. Failure to account for nutrient–C interactions can lead to substantial errors in predicting how ecosystems will respond to climate changes.
Increasing litterfall in lowland tropical forests could increase the amount of carbon released from the soil into the atmosphere.
In ‘Soil carbon release enhanced by increased tropical forest litterfall’, Emma Sayer et al. (2011) reported that tropical forests are a critical component of the global carbon cycle. They found that the efflux of CO2 derived from soil organic carbon was significantly increased by litter addition. The researchers predict that a future increase in litterfall of 30% with an increase in atmospheric CO2 concentrations of 150 ppm could release about 0.6 t C ha−1 yr−1 from the soil. Their response to environmental change will play a key role in determining future concentrations of atmospheric carbon dioxide (CO2). They show that experimentally increasing litterfall in a lowland tropical forest enhanced carbon release from the soil.
Tropical forests recover faster from natural and human disturbances than those in other parts of the world.
Lydia Cole et al. (2014) reported on recovery and resilience of tropical forests after disturbance. The time taken for forested tropical ecosystems to re-establish post-disturbance is of widespread interest. Disturbance events are defined as perturbations that result in a sharp loss of forest pollen in the palynological record and subsequent gain. Results demonstrate that the majority of past disturbances were caused by forest clearing, followed by climatic changes and large infrequent events. These patterns have emerged, none of the locations or disturbance categories proved significantly different to each other in their SRD. This suggests forests in Central America have not experienced considerably more disturbance events per millennium than the other tropical regions in the past.
The analysis involved 71 studies. Some of the conclusions look to corroborate previous studies in this area: “Despite being labelled as large infrequent disturbances, these perturbations are also the type of perturbation that has occurred with the greatest frequency in the past. This further supports one of the main conclusions of this study that these ecosystems recover most rapidly after exposure to natural disturbances,” Cole posited. However, “Future work would extend understanding of the causal reasons underlying this study’s findings and provide further knowledge on the ecology of tropical forests, as well as information for application in ecosystem management and restoration,” concede the authors. They recommend that findings provide valuable insights for the sustainable use of tropical forest resources. For the development of successful ecosystem service markets, trading in, for example, carbon credits resulting from effective forest restoration, is sorely lacking.
Scientists are increasingly concerned about the impact of climate change on trees in moist tropical forests, but little is known about the drivers, mechanisms and interactions that may underlie increasing mortality.
Nate McDowell et al. (2018) described drivers and mechanisms of tree mortality in moist tropical forests. Moist tropical forests are the largest terrestrial carbon sink in the world and house the majority of Earth’s terrestrial biodiversity. Shading is presumed to be the dominant driver of the high mortality rates of seedlings and understory plants. The researchers propose that drivers kill trees via the mechanisms of carbon starvation and hydraulic failure. The mechanisms of the interactions between shade, herbivory, biotic agents and the physiological mechanisms of carbon starvation and hydraulic failure within the ultimate mortality process is poorly known.
Shading in light-limited MTFs has been associated with carbon starvation in four species of angiosperm. The dichotomy between the low-light environment and the highlight environment has had a distinct impact on the evolutionary strategy of species.
There is a growing need for operational biodiversity mapping methods to quantify and to assess the impact of climate change, habitat alteration, and human activity on ecosystem composition and function.
Jean-Baptiste Féret and Gregory Asner (2014) described mapping tropical forest canopy diversity using high-fidelity imaging spectroscopy. There is a growing need for operational biodiversity mapping methods to quantify and to assess the impact of climate change, habitat alteration, and human activity on ecosystem composition and function. They present an original method for the estimation of α‐ and β‐diversity of tropical forests based on high‐fidelity imaging spectroscopy. Airborne maps of H′ were highly correlated with field α‐diversity estimates.
Soil oxygen (O2) concentrations in humid tropical forests fluctuate over hours to weeks, according to a time series of soil and greenhouse gas measurements.
Daniel Liptzin et al. (2010) report that oxygen is a fundamental driver of biogeochemical cycling on Earth. The mean O2 concentration in Bisley was significantly higher than in the cloud forest. The effects of O2 depletion on the biogeochemistry of flooded soils have been well documented. Methane concentrations were on average more than three orders of magnitude larger in the cloud forest soils than in Bisley. The heterogeneous redox environment in these wet tropical forest soils presents challenges to predicting biogeochemistry, especially in the Bisley site.
Soil Oxygen and Greenhouse Gases 173 data were collected hourly using a data logger and multiplexer. Trace gas sampling occurred weekly in Bisley and biweekly in the cloud forest. Some of the researchers’ conclusions look to offer an alternative view to previous research in this field: “Theory would predict 1:1 M relationship of CO2 production and O2 consumption during organic C mineralization to CO2 with O2 as the dominant electron acceptor. This is not always observed in the field, however, and was not observed here,” Liptzin suggested.
The role of climate on the current and future distribution of humid tropical forests has been the subject of a number of studies, but these are the first of their kind to address the issue on a much finer scale.
Przemyslaw Zelazowski et al. (2010) reported on changes in the potential distribution of humid tropical forests on a warmer planet. The future of tropical forests has become one of the iconic issues in climate-change science. This study focuses on the role of climate on the current and future distribution of humid tropical forests (HTFs).
Tropical forests are more responsive to climate change than recovery to past disturbances.
Carolyn and Brian Enquist (2010) report that disentangling the relative roles of biotic and abiotic forces influencing forest structure, function, and local community composition continues to be an important goal in ecology. They assess the relative role of past disturbance and local climatic change in the form of increased drought in driving forest dynamics. Over relatively short time scales, community composition and the functional dominance may be more responsive to climate change than recovery to past disturbances. Quantifying proportional functional composition offers a basis for a predictive framework for how the structure, and diversity of tropical forests will respond to global change.
Seasonally dry tropical forests (SDTF) are located in regions with alternating wet and dry seasons, with dry seasons that last several months or more.
Kara Allen et al. (2017) described how will seasonally dry tropical forests be sensitive or resistant to future changes in rainfall regimes?. Dry tropical forests once occupied vast amounts of tropical lands. Up to 40% of all tropical forest was dry before large-scale deforestation. Drier tropical forests may be slower to recover from intense or prolonged droughts. Most SDTF species have evolved strategies such as leaf deciduousness to tolerate or avoid severe seasonal drought. Increased inter-annual rainfall variability, greater intervals between extremely wet and dry years, and a decline in rainfall predicted for SDTF could influence the relative performance of species with different leaf habits and traits.
Dendrochronology and tree ring analysis can provide evidence of the coupling between tree growth and climate for many tropical dry forest species. For example, a 60þ year record of seven diverse species from a dry forest in Bolivia found positive correlations between ring width and precipitation. Aspects of the authors’ findings look to contrast with preceding studies in this area: “We define drought sensitivity as changes in biological variables, states, processes, or species distributions that occur when rainfall is at or below the lower limits of what has been previously observed. By contrast, ecological resilience implies the ability to recover from changes in a relatively short amount of time,” Allen said. Allen and colleagues argue that future research should focus on geographically comparative studies and well-replicated drought experiments that can provide empirical evidence to improve simulation models used to forecast SDTF responses to future climate change.
A study of a transitional forest in the Brazilian Amazon has revealed that invasive C 4 grasses increase fire frequency in response to increasing pasture and native grass invasion.
A research team at the Woods Hole Research Center led by Divino Silvério (2013) described testing the Amazon savannization hypothesis. In many parts of the world, tropical forest–savannah boundaries shift in response to changing climate and disturbance regimes. They first measured grass invasion from the edge into the forest interior annually from 2004 to 2011 in the control, B1yr and B3yr. In 2012, they complemented this measurement by mapping the presence of grasses within 250 m of the forest edge at a resolution of 5 Â 5 m. In August 2010, fine fuel loads along the forest edge were highest in areas dominated by exotic grasses and lowest in mixed vegetation types. While they sowed seeds of B. decumbens in quantities considered high enough to overcome seed limitation, this African pasture grass covered only a small fraction of the experimental subplots.
The average annual precipitation of 1700 mm falls predominantly during the October–May rainy season. This forest is less diverse than most wet and moist forests, with only 97 woody species ha21 greater than 10 cm diameter at breast height. However, “This spatial pattern was expected given that pastures bordered the experimental plots for more than 20 years. Apparently, while wind, animals, insects and human visitors dispersed some grass seeds into the forest interior, dispersal was limited,” say the investigators. The authors have provided data and code at: http://dx.doi.org/10.1098/rstb.2012.0427, http://rstb.royalsocietypublishing.org.
Tropical forests are under increasing threat from a range of anthropogenic factors.
S. Wright (2010) reported on the future of tropical forests. Five anthropogenic drivers–land use change, wood extraction, hunting, atmospheric change, climate change–will largely determine the future of tropical forests. Commercial logging is shifting rapidly from Southeast Asia to Africa and South America. Pantropical rates of net deforestation are declining even as secondary and logged forests increasingly replace old‐growth forests. Contemporary land use change includes deforestation and natural forests regenerating on abandoned land.
The role of phosphorus in controlling the productivity of tropical ecosystems and global climate change has been explored in a new carbon-climate model.
X. Yang et al. (2013) report that high rates of CO2 exchange between the tropical ecosystem and the atmosphere suggest that changes in tropical ecosystems can affect the pace of atmospheric CO2 increase and climate change. In spite of the likely significance of tropical ecosystems to the global carbon cycle, tropical carbon cycle processes and the feedbacks to the climate system remain critically uncertain. The model underestimated the increase in plant production associated with the addition of N and P to- Generally the introduction of P limitation (CLM-CNP versus gether at the N-limited site) improved the modeled NPP at the Amazon forest of P availability. Increased SOM and production tions explicitly in CLM help improve the understanding of organic acid.
The analysis involved 34 separate fertilization studies. Discussing possible shortcomings, “Sorption hanced when increased SOM under elevated CO2 occupies and desorption may be affected by soil pH and redox flucmore of the sorption sites on soil minerals,” they observe. They advocate that future studies will evaluate how changes in land cover and land use affect nutrient dynamics and C uptake in tropical forests. A comprehensive sensitivity analysis would help us to improve the understanding of the model and quantify model uncertainties. Data and code are available from: http://www.biogeosciences.net/11/1667/2014/bg-11-1667-2014-supplement.pdf.
Molly Cavaleri et al. (2015) report that tropical forests represent one of the planet’s most active biogeochemical engines. The authors discuss the three abiotic variables associated with climatic change that are most likely to affect C cycling of plants and soil in tropical forests. Priority should be to start with field warming experiments investigating the temperature responses of the most biogeochemically-active components of the system. Given the large amounts of C tropical forests store and cycle, investigations of tropical forest response to these environmental drivers will be critical for the understanding of future global-scale climate.
Tropical forests are on a trajectory to become altered ecosystems, with the degree of alteration dependent on the intensity and duration of human-induced pressures.
Yadvinder Malhi et al. (2014) reported in ‘Tropical Forests in the Anthropocene’ that the start of the Anthropocene is open to debate (3) with some arguments for a start at the dawn of agriculture (5,000–10,000 years ago) or even with the Pleistocene megafaunal extinctions. Key facets include deforestation driven by agricultural expansion, timber and wood extraction, the loss of fauna that maintain critical ecological connections, the spread of fire, landscape fragmentation, and climate change. Tropical forests across the world are changing owing to a diverse array of drivers, including clearance and fragmentation for agriculture.
The researchers have provided data and code at: http://dx.doi.org/10.6084/m9.figshare.1018762.
References
Guan, K., Pan, M., Li, H., Wolf, A., Wu, J., Medvigy, D., … Lyapustin, A. I. (2015). Photosynthetic seasonality of global tropical forests constrained by hydroclimate. Nature Geoscience, 8(4), 284–289. doi:10.1038/ngeo2382 [Link]
Phillips, O. L., van der Heijden, G., Lewis, S. L., López-González, G., Aragão, L. E. O. C., Lloyd, J., … Dávila, E. A. (2010). Drought-mortality relationships for tropical forests. New Phytologist, 187(3), 631–646. doi:10.1111/j.1469-8137.2010.03359.x [Link]
Werneck, F. P., Costa, G. C., Colli, G. R., Prado, D. E., & Sites Jr, J. W. (2010). Revisiting the historical distribution of Seasonally Dry Tropical Forests: new insights based on palaeodistribution modelling and palynological evidencegeb. Global Ecology and Biogeography, 20(2), 272–288. doi:10.1111/j.1466-8238.2010.00596.x [Link]
Leite, Y. L. R., Costa, L. P., Loss, A. C., Rocha, R. G., Batalha-Filho, H., Bastos, A. C., … Pardini, R. (2016). Neotropical forest expansion during the last glacial period challenges refuge hypothesis. Proceedings of the National Academy of Sciences, 113(4), 1008–1013. doi:10.1073/pnas.1513062113 [Link]
Bouskill, N. J., Lim, H. C., Borglin, S., Salve, R., Wood, T. E., Silver, W. L., & Brodie, E. L. (2012). Pre-exposure to drought increases the resistance of tropical forest soil bacterial communities to extended drought. The ISME Journal, 7(2), 384–394. doi:10.1038/ismej.2012.113 [Link]
Huang, Y., Chen, Y., Castro-Izaguirre, N., Baruffol, M., Brezzi, M., Lang, A., … Yang, X. (2018). Impacts of species richness on productivity in a large-scale subtropical forest experiment. Science, 362(6410), 80–83. doi:10.1126/science.aat6405 [Link]
Berenguer, E., Ferreira, J., Gardner, T. A., Aragão, L. E. O. C., De Camargo, P. B., Cerri, C. E., … Barlow, J. (2014). A large-scale field assessment of carbon stocks in human-modified tropical forests. Global Change Biology, 20(12), 3713–3726. doi:10.1111/gcb.12627 [Link]
Feeley, K. J., Davies, S. J., Perez, R., Hubbell, S. P., & Foster, R. B. (2011). Directional changes in the species composition of a tropical forest. Ecology, 92(4), 871–882. doi:10.1890/10-0724.1 [Link]
Townsend, A. R., Cleveland, C. C., Houlton, B. Z., Alden, C. B., & White, J. W. (2011). Multi‐element regulation of the tropical forest carbon cycle. Frontiers in Ecology and the Environment, 9(1), 9–17. doi:10.1890/100047 [Link]
Sayer, E. J., Heard, M. S., Grant, H. K., Marthews, T. R., & Tanner, E. V. J. (2011). Soil carbon release enhanced by increased tropical forest litterfall. Nature Climate Change, 1(6), 304–307. doi:10.1038/nclimate1190 [Link]
Cole, L. E. S., Bhagwat, S. A., & Willis, K. J. (2014). Recovery and resilience of tropical forests after disturbance. Nature Communications, 5(1). doi:10.1038/ncomms4906 [Link]
McDowell, N., Allen, C. D., Anderson-Teixeira, K., Brando, P., Brienen, R., Chambers, J., … Xu, X. (2018). Drivers and mechanisms of tree mortality in moist tropical forests. New Phytologist, 219(3), 851–869. doi:10.1111/nph.15027 [Link]
Féret, J.-B., & Asner, G. P. (2014). Mapping tropical forest canopy diversity using high-fidelity imaging spectroscopy. Ecological Applications, 24(6), 1289–1296. doi:10.1890/13-1824.1 [Link]
Liptzin, D., Silver, W. L., & Detto, M. (2010). Temporal Dynamics in Soil Oxygen and Greenhouse Gases in Two Humid Tropical Forests. Ecosystems, 14(2), 171–182. doi:10.1007/s10021-010-9402-x [Link]
Zelazowski, P., Malhi, Y., Huntingford, C., Sitch, S., & Fisher, J. B. (2011). Changes in the potential distribution of humid tropical forests on a warmer planet. Philosophical Transactions of the Royal Society A: Mathematical, Physical and Engineering Sciences, 369(1934), 137–160. doi:10.1098/rsta.2010.0238 [Link]
Enquist, B. J., & Enquist, C. A. F. (2010). Long-term change within a Neotropical forest: assessing differential functional and floristic responses to disturbance and drought. Global Change Biology, 17(3), 1408–1424. doi:10.1111/j.1365-2486.2010.02326.x [Link]
Allen, K., Dupuy, J. M., Gei, M. G., Hulshof, C., Medvigy, D., Pizano, C., … Powers, J. S. (2017). Will seasonally dry tropical forests be sensitive or resistant to future changes in rainfall regimes? Environmental Research Letters, 12(2), 023001. doi:10.1088/1748-9326/aa5968 [Link]
Silvério, D. V., Brando, P. M., Balch, J. K., Putz, F. E., Nepstad, D. C., Oliveira-Santos, C., & Bustamante, M. M. C. (2013). Testing the Amazon savannization hypothesis: fire effects on invasion of a neotropical forest by native cerrado and exotic pasture grasses. Philosophical Transactions of the Royal Society B: Biological Sciences, 368(1619), 20120427. doi:10.1098/rstb.2012.0427 [Link]
Wright, S. J. (2010). The future of tropical forests. Annals of the New York Academy of Sciences, 1195(1), 1–27. doi:10.1111/j.1749-6632.2010.05455.x [Link]
Yang, X., Thornton, P. E., Ricciuto, D. M., & Post, W. M. (2013). The role of phosphorus dynamics in tropical forests – a modeling study using CLM-CNP. doi:10.5194/bgd-10-14439-2013 [Link]
Cavaleri, M. A., Reed, S. C., Smith, W. K., & Wood, T. E. (2015). Urgent need for warming experiments in tropical forests. Global Change Biology, 21(6), 2111–2121. doi:10.1111/gcb.12860 [Link]
Malhi, Y., Gardner, T. A., Goldsmith, G. R., Silman, M. R., & Zelazowski, P. (2014). Tropical Forests in the Anthropocene. Annual Review of Environment and Resources, 39(1), 125–159. doi:10.1146/annurev-environ-030713-155141 [Link]